Microbial biogeography: putting microorganisms on the map
Jennifer B. Hughes Martiny, Brendan J. M. Bohannan, James Brown, Robert K Colwell, Jed A. Fuhrman, Jessica L. Green, M. Claire Horner-Devine, Matthew Kane, Jennifer Adams Krumins, Cheryl R. Kuske, Peter J. Morin, Shahid Naeem, Lise Øvreås, Anna-Louise Reysenbach, V. H. Smith, James T Staley
NATURE REVIEWS - MICROBIOLOGY - FEBRUARY 2006 - VOLUME 4 -pp 102 - 112
Abstract
We review the biogeography of microorganisms in light of the biogeography of macroorganisms. A large body of research supports the idea that free-living microbial taxa exhibit biogeographic patterns. Current evidence confirms that, as proposed by the Baas-Becking hypothesis, ‘the environment selects’ and is, in part, responsible for spatial variation in microbial diversity. However, recent studies also dispute the idea that ‘everything is everywhere’. We also consider how the processes that generate and maintain biogeographic patterns in macroorganisms could operate in the microbial world.
Biogeography is the study of the distribution of biodiversity over space and time. It aims to reveal where organisms live, at what abundance, and why. The study of biogeography offers insights into the mechanisms that generate and maintain diversity, such as speciation, extinction, dispersal and species interactions1 . Since the eighteenth century, biologists have investigated the geographic distribution of plant and animal diversity. More recently, the geographic distributions of microorganisms have been examined. Genetic methodologies have revealed that past culture-based studies missed most microbial diversity2–4, and have allowed recent studies to sample microbial diversity more deeply and widely than ever before5,6. Microbial biogeography stands to benefit tremendously from these advances, although there is still debate as to whether microorganisms exhibit any biogeographic patterns7–10
Although traditionally confined to separate academic disciplines, ecologists who study microorganisms and those who study macroorganisms have been interacting more often in recent years. Indeed, this article is a result of a National Center for Ecological Analysis and Synthesis (NCEAS) working group composed of scientists from both specialties. Our goal here is to review what is known regarding the biogeography of microorganisms in light of that of macroorganisms. This inquiry is not driven by the expectation that microorganisms simply follow the patterns of macroorganisms, but rather by the fact that the biogeography of macroorganisms is much better studied. Furthermore, micro- and macroorganisms are often involved in intimate associations that affect each other’s geographic distributions11,12. Therefore, a logical first hypothesis is that the biogeography of microorganisms is similar to the biogeography of macroorganisms. To the extent that microorganisms conform to the relationships documented for macroorganisms, they will extend the generality of empirical patterns and support mechanistic hypotheses that all living entities share universal attributes. Alternatively, if microorganisms can be shown to represent clear exceptions to the biogeographic patterns of plants and animals, then this will call attention to unique features of microbial life that have influenced the generation and maintenance of its diversity.
As an initial step towards distinguishing between these hypotheses, we suggest a framework for investigating whether microbial assemblages differ in different places and the extent to which this spatial variation is due to contemporary environmental factors and historical contingencies. We then discuss the mechanistic processes that generate and maintain biogeographic patterns in macroorganisms and consider their relevance to microbial biogeography
There is no universal definition of a ‘micro organism’. The term generally denotes members of the domains Bacteria and Archaea, as well as microscopic members of the domain Eukarya (for example, unicellular algae, some fungi and protists). For convenience, we further define a microorganism as having a mass of less than 10–5 g and a length of less than 500 µm.
We do not consider the question of whether viruses have bio geography, as their biology adds further complications and, in most cases, far less is known about their distribution than that of other microorganisms (for a recent discussion see REF. 13).
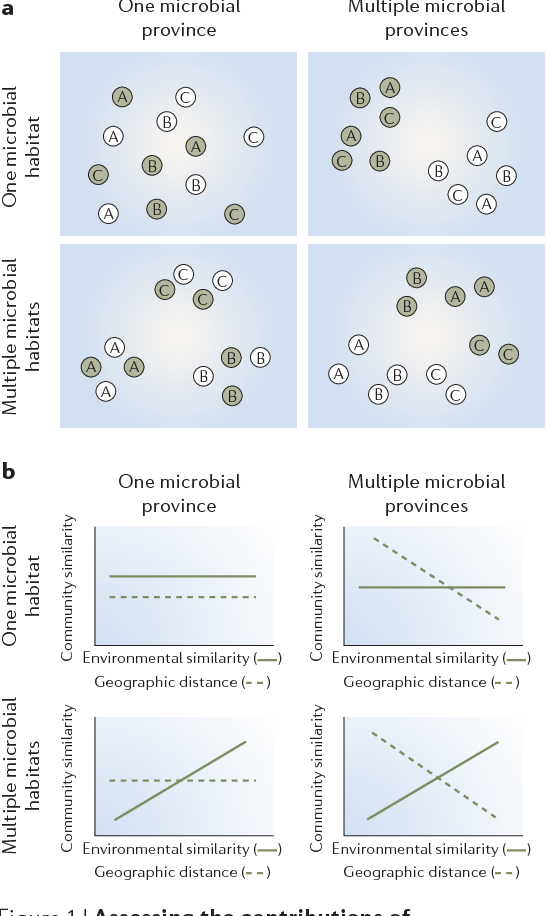
Figure 1 | Assessing the contributions of environmental and historical effects on microbial biogeography. Four alternative hypotheses about environmental and historical influences on communities and the general results that would support them, using (a) samples (shown in circles) from discrete predefined habitat types (A, B and C) and locations (green versus white) or (b) samples from continuous habitat variables and geographic distances. The axes in (a) are dimensionless; samples that contain similar assemblages are mapped closer to one another relative to pairs of samples with different assemblages. In b, lack of a correlation between environmental similarity or geographic distance and biotic similarity (BOX 1) indicates no biogeographic patterning. Alternatively, to the extent that environmental and historical factors have influenced the assemblages sampled, biotic similarity should be correlated with environmental similarity and geographic distance, respectively. Standard correlation tests are not appropriate to distinguish between these hypotheses because of non-independence; therefore, randomization tests such as a bootstrapped regression analysis25 or Mantel tests87,88 are required. Further tests, such as a partial Mantel test, can disentangle the effects of geographic distance versus environment on assemblage composition89.
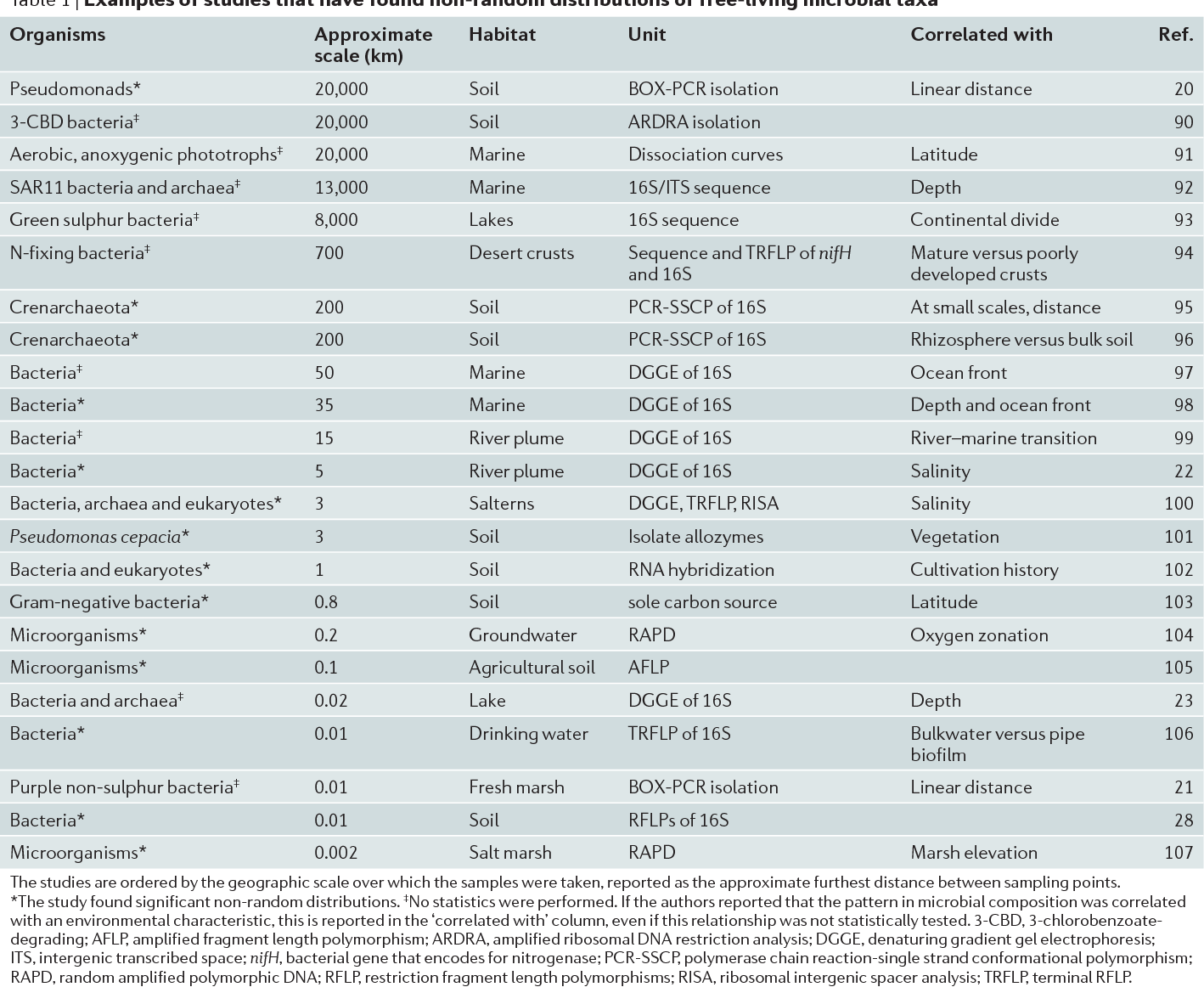
Table 1 | Examples of studies that have found non-random distributions of free-living microbial taxa
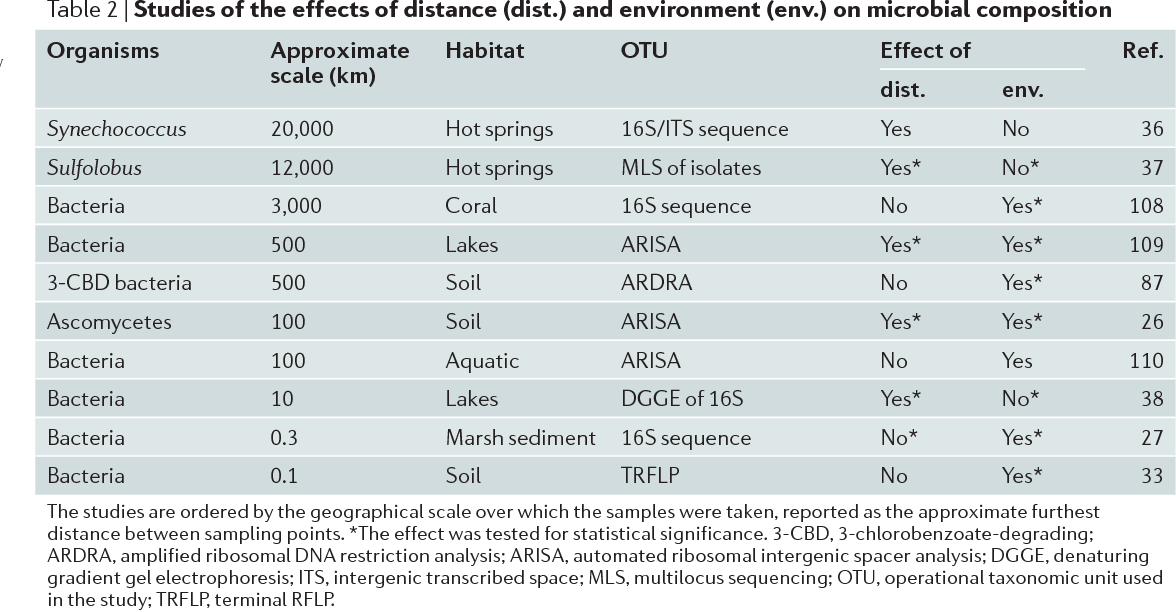
Table 2 | Studies of the effects of distance (dist.) and environment (env.) on microbial composition
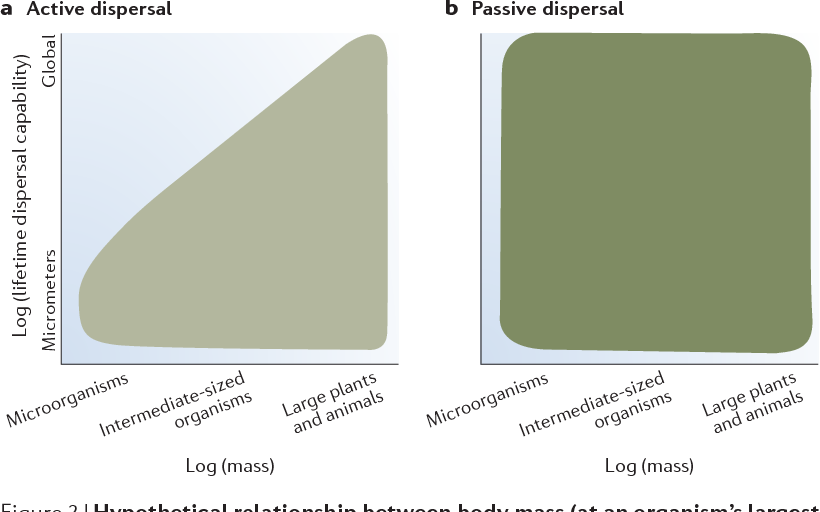
Figure 2 | Hypothetical relationship between body mass (at an organism’s largest life stage) and lifetime dispersal capability. The relationship varies depending on whether the organism disperses actively or passively (by its own propulsion). The range of active (a) dispersal is a subset of the range of passive (b) dispersal. It is convenient to think of the log(mass) axis as representing three qualitative groups: first, microorganisms, which span about 8 orders of magnitude from bacteria to eukaryotic algae and protozoa (10–13–10–5 g); second, large plants and animals, which span about 8 orders of magnitude from herbs and small vertebrates to whales and trees (101–109 g); and third, intermediatesized organisms, which span the intervening 6 or so orders of magnitude and include the small metazoans, such as nematodes, annelids and arthropod
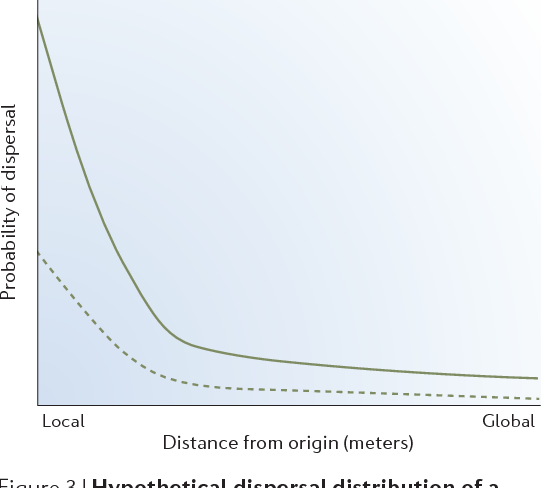
Figure 3 | Hypothetical dispersal distribution of a typical passively dispersed macroorganism. Population density influences the probability that an individual from that population will disperse over very long distances (solid line). For taxa with relatively low densities (dashed line), dispersal might be effectively restricted, even though long-distance movement is theoretically possible. Based on REF. 41.
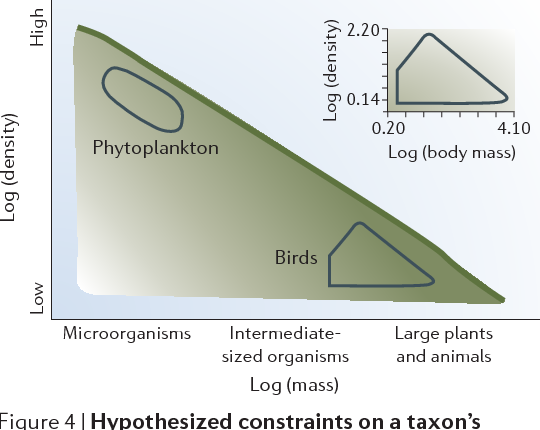
Figure 4 | Hypothesized constraints on a taxon’s population density in a given body-size class. The thick green line on the diagonal is a known physiological constraint. The gradient in shading from the diagonal to the bottom-left corner represents the idea that fewer taxa are thought to fall in the bottom left of the figure; however, we hypothesize that some taxa do fall in this region. The inset plots log (body mass, g) of North American birds versus log (population density, individuals per route). The data set falls within a well-defined quadrilateral with a constant minimum density and a maximum density for birds of an intermediate size. Individual data points are not shown. The outline of these data is also sketched on the constraint figure. The approximate range of marine phytoplankton data from Li48 is also sketched (assuming that cell volume is proportional to body mass). The X-axis categories are defined in FIG. 2. Inset adapted with permission from REF. 43 © (1987) University of Chicago.
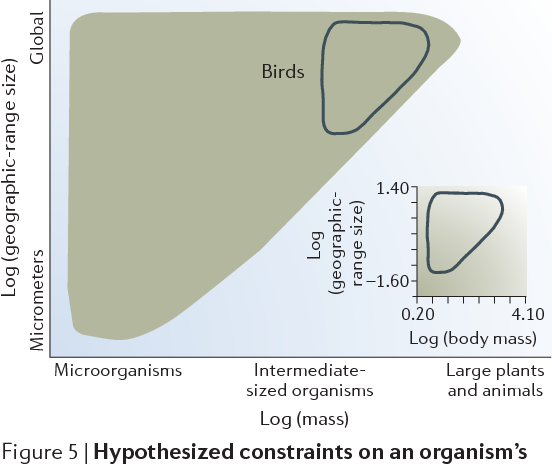
Figure 5 | Hypothesized constraints on an organism’s geographic-range size for a given body mass. The inset graph is log (body size, g) versus log (geographic-range size, 106 km2) for terrestrial bird species of North America. Individual data points are not shown. The combined data set forms an approximate triangle. The outline of these data is also sketched on the constraint figure. The X-axis categories are defined in FIG. 2. Inset adapted with permission from REF. 43 © (1987) University of Chicago.
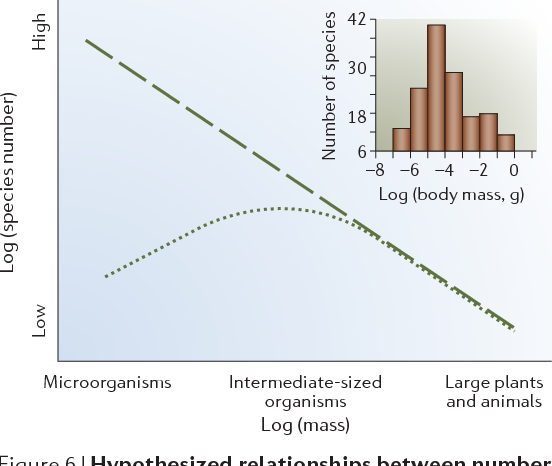
Figure 6 | Hypothesized relationships between number of species and body mass. For larger macroorganisms (approximately larger than insects), it is clear that the number of species increases as body mass decreases. For smaller macroorganisms and microorganisms, the number of species might continue to increase (dashed line) or begin to decrease (dotted line) as body mass decreases. The inset plots the number of invertebrate species by log(mass) on Marion Island64. The X-axis categories are defined in FIG. 2. Inset reproduced with permission from REF. 64 © (2001) National Academy of Sciences, USA.
1. Brown, J. H. & Lomolino, M. V. Biogeography (Sinauer, Sunderland, 1998). A definitive textbook on the biogeography of macroorganisms; has just been updated in a 2005 edition.
2. Ward, D. M., Weller, R. & Bateson, M. M. 16S ribosomal-RNA sequences reveal numerous uncultured microorganisms in a natural community. Nature 345, 63–65 (1990).
3. Øvreås, L. Population and community level approaches for analysing microbial diversity in natural environments. Ecol. Lett. 3, 236–251 (2000).
4. Floyd, M. M., Tang, J., Kane, M. & Emerson, D. Captured diversity in a culture collection: case study of the geographic and habitat distributions of environmental isolates held at the American type culture collection. Appl. Environ. Microbiol. 71, 2813–2823 (2005).
5. Schloss, P. D. & Handelsman, J. Status of the microbial census. Microbiol. Mol. Biol. Rev. 68, 686–691 (2004).
6. Venter, J. C. et al. Environmental genome shotgun sequencing of the Sargasso Sea. Science 304, 66–74 (2004).
7. Fenchel, T., Esteban, G. F. & Finlay, B. J. Local versus global diversity of microorganisms: cryptic diversity of ciliated protozoa. Oikos 80, 220–225 (1997).
8. Staley, J. T. Biodiversity: are microbial species threatened? Curr. Opin. Biotechnol. 8, 340–345 (1997).
9. Finlay, B. J. Global dispersal of free-living microbial eukaryote species. Science 296, 1061–1063 (2002). A summary of the arguments for why microbial eukaryotes might not be restricted by geographic barriers.
10. Hedlund, B. P. & Staley, J. T. Microbial endemism and biogeography. In Microbial Diversity and Bioprospecting (ed. Bull, A. T.) (ASM, Washington DC, 2003).
11. Anagnostakis, S. Chestnut blight: the classical problem of an introduced pathogen. Mycologia 79, 23–27 (1987).
12. Falush, D. et al. Traces of human migrations in Helicobacter pylori populations. Science 299, 1582–1585 (2003).
13. Breitbart, M. & Rohwer, F. Here a virus, there a virus, everywhere the same virus? Trends Microbiol. 13, 278–284 (2005).
14. de Candolle, A. P. Essai Elementaire de Geographie Botanique (F. G. Levrault, Paris, 1820).
15. Beijerinck, M. W. De infusies en de ontdekking der backteriën. In Jaarboek van de Koninklijke Akademie van Wetenschappen (Müller, Amsterdam, 1913).
16. Baas-Becking, L. G. M. Geobiologie of Inleiding Tot de Milieukunde (Van Stockkum & Zoon, The Hague, 1934).
17. Zhang, N. & Blackwell, M. Population structure of dogwood anthracnose fungus. Phytopathology 92, 1276–1283 (2002).
18. Bala, A., Murphy, P. & Giller, K. E. Distribution and diversity of rhizobia nodulating agroforestry legumes in soils from three continents in the tropics. Mol. Ecol. 12, 917–929 (2003).
19. Papke, R. T. & Ward, D M. The importance of physical isolation to microbial diversification. FEMS Microbiol. Ecol. 48, 293–303 (2004). A recent review discussing how physical isolation might affect prokaryote evolution.
20. Cho, J. C. & Tiedje, J. M. Biogeography and degree of endemicity of fluorescent Pseudomonas strains in soil. Appl. Environ. Microbiol. 66, 5448–5456 (2000). One of the first examples of relating the genetic similarity of a free-living bacterial assemblage with geographic distance, using fluorescent Pseudomonas isolates.
21. Oda, Y., Star, B., Huisman, L. A., Gottschal, J. C. & Forney, L. J. Biogeography of the purple nonsulfur bacterium Rhodopseudomonas palustris. Appl. Environ. Microbiol. 69, 5186–5191 (2003).
22. Crump, B. C., Hopkinson, C. S., Sogin, M. L. & Hobbie, J. E. Microbial biogeography along an estuarine salinity gradient: combined influences of bacterial growth and residence time. Appl. Environ. Microbiol. 70, 1494–1505 (2004).
23. Øvreås, L., Forney, L., Daae, R. L. & Torsvik, V. Distribution of bacterioplankton in meromictic Lake Sælenvannet, as determined by denaturing gradient gel electrophoresis of PCR-amplified gene fragments coding for 16S rRNA. Appl. Environ. Microbiol. 63, 3367–3373 (1997).
24. Schwalbach, M. S., Hewson, I. & Fuhrman, J. A. Viral effects on bacterial community composition in marine plankton microcosms. Aquat. Microb. Ecol. 34, 117–127 (2004).
25. Rosenzweig, M. L. Species Diversity in Space and Time. (Cambridge University Press, Cambridge, 1995).
26. Green, J. L. et al. Spatial scaling of microbial eukaryote diversity. Nature 432, 747–750 (2004). Along with reference 27, this study uses distance–decay curves to demonstrate nonrandom bacterial-assemblage distributions; the studies then test whether the non-random distributions are due to isolation by distance and/or local environmental conditions.
27. Horner-Devine, M. C., Lage, M., Hughes, J. B. & Bohannan, B. J. M. A taxa–area relationship for bacteria. Nature 432, 750–753 (2004).
28. Noguez, A. M. et al. Microbial macroecology: highly structured prokaryotic soil assemblages in a tropical deciduous forest. Glob. Ecol. Biogeogr. 14, 241–248 (2005).
29. Bell, T. et al. Larger islands house more bacterial taxa. Science 308, 1884 (2005).
30. Smith, V. H. et al. Phytoplankton species richness scales consistently from laboratory microcosms to the world’s oceans. Proc. Natl Acad. Sci. USA 102, 4393–4396 (2005).
31. Cam, E. et al. Disentangling sampling and ecological explanations underlying species–area relationships. Ecology 84, 1118–1130 (2002). 32. Colwell, R. K., Mao, C. X. & Chang, J. Interpolating, extrapolating, and comparing incidence-based species accumulation curves. Ecology 85, 2717–2727 (2004).
33. Kuske, C. R. et al. Comparison of soil bacterial communities in rhizospheres of three plant species and the interspaces in an arid grassland. Appl. Environ. Microbiol. 68, 1854–1863 (2002).
34. Yannarell, A. C. & Triplett, E. W. Within- and between-lake variability in the composition of bacterioplankton communities: investigations using multiple spatial scales. Appl. Environ. Microbiol. 70, 214–223 (2004).
35. Legendre, P. & Legendre, L. Numerical Ecology 2nd edn (Elsevier, Amsterdam, 1998).
36. Papke, R. T., Ramsing, N. B., Bateson, M. M. & Ward, D. M. Geographical isolation in hot spring cyanobacteria. Environ. Microbiol. 5, 650–659 (2003).
37. Whitaker, R. J., Grogan, D. W. & Taylor, J. W. Geographic barriers isolate endemic populations of hyperthermophilic Archaea. Science 301, 976–978 (2003). Already a classic microbial biogeography study, it considers both the effects of spatial isolation and contemporary environmental parameters on hotspring Sulfolobus assemblages within and across continents.
38. Reche, I., Pulido-Villena, E., Morales-Baquero, R. & Casamayor, E. O. Does ecosystem size determine aquatic bacterial richness? Ecology 86, 1715–1722 (2005).
39. Brown, J. H., West, G. B. & Enquist, B. J. Scaling in biology: patterns and processes, causes and consequences. In Scaling in Biology (eds Brown, J. H. & West, G. B.) 1–24 (Oxford University Press, Oxford, 2000). A comprehensive introduction to allometric patterns in biology.
40. Bell, G. The distribution of abundance in neutral communities. Am. Nat. 155, 606–617 (2000).
41. Hubbell, S. P. The Unified Neutral Theory of Biodiversity and Biogeography (Princeton University Press, Princeton, 2001).
42. Clark, J. S., Silman, M., Kern, R., Macklin, E. & HilleRisLambers, J. Seed dispersal near and far: patterns across temperate and tropical forests. Ecology 80, 1475–1494 (1999).
43. Brown, J. H. & Maurer, B. A. Evolution of species assemblages: effects of energetic constraints and species dynamics on the diversification of the North American avifauna. Am. Nat. 130, 1–17 (1987).
44. Morse, D. R., Stork, N. E. & Lawton, J. H. Species number, species abundance and body length relationships of arboreal beetles in Bornean lowland rain forest trees. Ecol. Entomol. 13, 25–37 (1988).
45. Lawton, J. H. Species richness and population dynamics of animal assemblages. Patterns in body size: abundance space. Philos. Trans. R. Soc. Lond. B Biol. Sci. 330, 283–291 (1990).
46. Siemann, E., Tilman, D. & Haarstad, J. Insect species diversity, abundance and body size relationships. Nature 380, 704–706 (1996).
47. Brown, J. H. Macroecology (Chicago University Press, Chicago, 1995).
48. Li, W. K. W. Macroecological patterns of phytoplankton in the northwestern North Atlantic Ocean. Nature 419, 154–157 (2002).
49. Roberts, M. S. & Cohan, F. M. Recombination and migration rates in natural populations of Bacillus subtilis and Bacillus mojavensis. Evolution 49, 1081–1094 (1995).
50. Stanley, S. M. Macroevolution: Pattern and Process (W. H. Freeman, San Francisco, 1979).
51. Paradis, E. Statistical analysis of diversification with species traits. Evolution 59, 1–12 (2005). 52. Lenski, R. E. & Travisano, M. Dynamics of adaptation and diversification: a 10,000-generation experiment with bacterial-populations. Proc. Natl Acad. Sci. USA 91, 6808–6814 (1994).
53. Rainey, P. B. & Travisano, M. Adaptive radiation in a heterogeneous environment. Nature 394, 69–72 (1998).
54. Manne, L. L., Brooks, T. M. & Pimm, S. L. Relative risk of extinction of passerine birds on continents and islands. Nature 399, 258–261 (1999).
55. Purvis, A., Gittleman, J. L., Cowlishaw, G. & Mace, G. M. Predicting extinction risk in declining species. Proc. R. Soc. Lond. B Biol. Sci. 267, 1947–1952 (2000).
56. Lawton, J. H. Range, Population abundance and conservation. Trends Ecol. Evol. 8, 409–413 (1993).
57. Silva, M. & Downing, J. A. Allometric scaling of minimal mammal densities. Conserv. Biol. 8, 732– 743 (1994).
58. Meretsky, V. J., Snyder, N. F. R., Beissinger, S. R., Clendenen, D. A. & Wiley, J. W. Demography of the California condor: implications for reestablishment. Conserv. Biol. 14, 957–967 (2000).
59. Finlay, B. J. & Clarke, K. J. Apparent global ubiquity of species in the protist genus Paraphysomonas. Protist 150, 419–430 (1999).
60. Massana, R., DeLong, E. F.& Pedrós-Alió, C. A few cosmopolitan phylotypes dominate planktonic archaeal assemblages in widely different oceanic provinces. Appl. Environ. Microbiol. 66, 1777–1787 (2000).
61. Lindström, E. S. & Leskinen, E. Do neighboring lakes share common taxa of bacterioplankton? Comparison of 16S rDNA fingerprints and sequences from three geographic regions. Microb. Ecol. 44, 1–9 (2002).
62. Brown, J. H., Stevens, G. C. & Kaufman, D. M. The geographic range: size, shape, boundaries, and internal structure. Annu. Rev. Ecol. Syst. 27, 597– 623 (1996).
63. May, R. M. How many species are there on Earth? Science 241, 1441–1449 (1988).
64. Gaston, K. J., Chown, S. L. & Mercer, R. D. The animal species-body size distribution of Marion Island. Proc. Natl Acad. Sci. USA 98, 14493–14496 (2001).
65. Dykhuizen, D. E. Santa Rosalia revisited: why are there so many species of bacteria? Antonie Van Leeuwenhoek 73, 25–33 (1998).
66. Avise, J. C. & Aquadro, C. F. A comparative summary of genetic distances in the vertebrates. Evol. Biol. 15, 151–185 (1982).
67. de Vargas, C., Norris, R., Zaninetti, L., Gibb, S. W. & Pawlowski, J. Molecular evidence of cryptic speciation in planktonic foraminifers and their relation to oceanic provinces. Proc. Natl Acad. Sci. USA 96, 2864–2868 (1999).
68. Cohan, F. M. What are bacterial species? Annu. Rev. Microbiol. 56, 457–487 (2002).
69. Coyne, J. A., Orr, H. A. & Futuyma, D. J. Do we need a new species concept? Syst. Zool. 37, 190–200 (1988).
70. Green, J. L. & Bohannan, B. J. M. Spatial scaling of microbial biodiversity in Scaling Biodiversity (eds Storch, D. & Marquet, P. A. & Brown, J. H.) (Cambridge University Press, Cambridge, 2006).
71. Martiny, J. B. H. & Field, D. Ecological perspectives on the sequenced genome collection. Ecol. Lett. (in the press).
72. Naeem, S., Thompson, L. J., Lawler, S. P., Lawton, J. H. & Woodfin, R. M. Declining biodiversity can alter the performance of ecosystems. Nature 368, 734– 737 (1994).
73. Hooper, D. U. &. Vitousek, P. M. The effects of plant composition and diversity on ecosystem processes. Science 277, 1302–1305 (1997).
74. Dukes, J. S. Biodiversity and invasibility in grassland microcosms. Oecologia 126, 563–568 (2001).
75. Treseder, K. K. & Vitousek, P. M. Potential ecosystemlevel effects of genetic variation among populations of Metrosideros polymorpha from a soil fertility gradient in Hawaii. Oecologia 126, 266–275 (2001).
76. McGrady-Steed, J., Harris, P. M. & Morin, P. J. Biodiversity regulates ecosystem predictability. Nature 390, 162–165 (1997).
77. Bell, T., Newman, J. A., Silverman, B. W., Turner, S. L. & Lilley, A. K. The contribution of species richness and composition to bacterial services. Nature 436, 1157–1160 (2005).
78. Naeem, S. & Li, S. B. Biodiversity enhances ecosystem reliability. Nature 390, 507–509 (1997).
79. van der Heijden, M. G. A. et al. Mycorrhizal fungal diversity determines plant biodiversity, ecosystem variability and productivity. Nature 396, 69–72 (1998).
80. Cavigelli, M. A. & Robertson, G. P. The functional significance of denitrifier community composition in a terrestrial ecosystem. Ecology 81, 1402–1414 (2000).
81. Horz, H.-P., Barbrook, A., Field, C. B. & Bohannan, B. J. M. Ammonia-oxidizing bacteria respond to multifactorial global change. Proc. Natl Acad. Sci. USA 101, 15136–15141 (2004).
82. Bull, A. T., ed. Microbial Diversity and Bioprospecting. (ASM Press, Washington DC, 2003).
83. Finlay, B. J., Esteban, G. F., Olmo, J. L. & Tyler, P. A. Global distribution of free-living microbial species. Ecography 22, 138–144 (1999).
84. Magurran, A. E. Ecological Diversity and Its Measurement (Princeton University Press, Princeton, 1988).
85. Chao, A., Chazdon, R. L., Colwell, R. K. & Shen, T. J. A new statistical approach for assessing similarity of species composition with incidence and abundance data. Ecol. Lett. 8, 148–159 (2005).
86. Ricketts, T. H. The matrix matters: effective isolation in fragmented landscapes. Am. Nat. 158, 87–99 (2001).
87. Mantel, N. The detection of disease clustering and a generalized regression approach. Cancer Res. 27, 209–220 (1967).
88. Borcard, D., Legendre, P. & Drapeau, P. Partialling out the spatial component of ecological variation. Ecology 73, 1045–1055 (1992).
89. Smouse, P. E., Long, J. C. & Sokal, R. R. Multiple regression and correlation extensions of the Mantel test of matrix correspondence. Syst. Zool. 35, 627– 632 (1986).
90. Fulthorpe, R. R., Rhodes, A. N. & Tiedje, J. M. High levels of endemicity of 3-chlorobenzoate-degrading soil bacteria. Appl. Environ. Microbiol. 64, 1620– 1627 (1998).
91. Schwalbach, M. S. & Fuhrman, J. A. Wide-ranging abundances of aerobic anoxygenic phototrophic bacteria in the world ocean revealed by epifluorescence microscopy and quantitative PCR. Limnol. Oceanogr. 50, 620–628 (2005).
92. Garcia-Martinez, J. & Rodriguez-Valera, F. Microdiversity of uncultured marine prokaryotes: the SAR11 cluster and the marine Archaea of Group I. Mol. Ecol. 9, 935–948 (2000).
93. Glaeser, J. & Overmann, J. Biogeography, evolution, and diversity of epibionts in phototrophic consortia. Appl. Environ. Microbiol. 70, 4821–4830 (2004).
94. Yeager, C. M. et al. Diazotrophic community structure and function in two successional stages of biological soil crusts from the Colorado plateau and Chihuahuan desert. Appl. Environ. Microbiol. 70, 973–983 (2004).
95. Sliwinski, M. K. & Goodman, R. M. Spatial heterogeneity of Crenarchaeal assemblages within mesophilic soil ecosystems as revealed by PCR-singlestranded conformation polymorphism profiling. Appl. Environ. Microbiol. 70, 1811–1820 (2004).
96. Sliwinski, M. K. & Goodman, R. M. Comparison of Crenarchaeal consortia inhabiting the rhizosphere of diverse terrestrial plants with those in bulk soil in native environments. Appl. Environ. Microbiol. 70, 1821–1826 (2004).
97. Riemann, L. & Middelboe, M. Stability of bacterial and viral community compositions in Danish coastal waters as depicted by DNA fingerprinting techniques. Aquat. Microb. Ecol. 27, 219–232 (2002).
98. Pinhassi, J. et al. Spatial variability in bacterioplankton community composition at the Skagerrak–Kattegat Front. Mar. Ecol. Prog. Ser. 255, 1–13 (2003). 99. Troussellier, M. et al. Bacterial activity and genetic richness along an estuarine gradient (Rhone River plume, France). Aquat. Microb. Ecol. 28, 13–24 (2002). 100. Casamayor, E. O. et al. Changes in archaeal, bacterial and eukaryal assemblages along a salinity gradient by comparison of genetic fingerprinting methods in a multipond solar saltern. Environ. Microbiol. 4, 338– 348 (2002).
101. McArthur, J. V., Kovacic, D. A. & Smith, M. H. Genetic diversity in natural populations of a soil bacterium across a landscape gradient. Proc. Natl Acad. Sci. USA 85, 9621–9624 (1988).
102. Buckley, D. H. & Schmidt, T. M. Diversity and dynamics of microbial communities in soils from agro-ecosystems. Environ. Microbiol. 5, 441–452 (2003).
103. Staddon, W. J., Trevors, J. T., Duchesne, L. C. & Colombo, C. A. Soil microbial diversity and community structure across a climatic gradient in western Canada. Biodivers. Conserv. 7, 1081–1092 (1998).
104. Franklin, R. B., Taylor, D. R. & Mills, A. L. The distribution of microbial communities in anaerobic and aerobic zones of a shallow coastal plain aquifer. Microb. Ecol. 38, 377–386 (1999).
105. Franklin, R. B. & Mills, A. L. Multi-scale variation in spatial heterogeneity for microbial community structure in an eastern Virginia agricultural field. FEMS Microbiol. Ecol. 44, 335–346 (2003).
106. Martiny, A. C., Jorgensen, T. M., Albrechtsen, H. J., Arvin, E. & Molin, S. Long-term succession of structure and diversity of a biofilm formed in a model drinking water distribution system. Appl. Environ. Microbiol. 69, 6899–6907 (2003).
107. Franklin, R. B., Blum, L. K., McComb, A. C. & Mills, A. L. A geostatistical analysis of small-scale spatial variability in bacterial abundance and community structure in salt marsh creek bank sediments. FEMS Microbiol. Ecol. 42, 71–80 (2002).
108. Rohwer, F., Seguritan, V., Azam, F. & Knowlton, N. Diversity and distribution of coral-associated bacteria. Mar. Ecol. Prog. Ser. 243, 1–10 (2002).
109. Yannarell, A. C. & Triplett, E. W. Geographic and environmental sources of variation in lake bacterial community composition. Appl. Environ. Microbiol. 71, 227–239 (2005).
110. Hewson, I. & Fuhrman, J. A. Richness and diversity of bacterioplankton species along an estuarine gradient in Moreton Bay, Australia. Appl. Environ. Microbiol. 70, 3425–3433 (2004).
Full Text
Or
Or
ليست هناك تعليقات:
إرسال تعليق